Nutrición mineral de líneas de frijol bajo clorosis férrica
DOI:
https://doi.org/10.29312/remexca.v14i2.3419Palabras clave:
deficiencia de hierro, estrategia I, estrategia II, estrés por hierro, nutrición vegetalResumen
La deficiencia de hierro existe en casi todos los cultivos del mundo y la habilidad para absorber el hierro varía ampliamente entre especies de plantas. Existen grupos de plantas que se caracterizan por su capacidad para crecer en suelos con baja disponibilidad de hierro, denominadas Fe-eficientes. En este experimento se cultivaron en invernadero plantas de frijol, en una solución nutritiva con concentraciones subóptimas y óptimas de Fe. El objetivo fue evaluar los mecanismos de tolerancia, concentración y distribución del Fe en líneas de frijol. Se establecieron seis líneas de frijol (tres tolerantes y tres susceptibles a deficiencia de hierro). Se evaluó la concentración nutrimental y unidades SPAD en hojas jóvenes y raíces, volumen radical y materia seca. Los resultados obtenidos determinaron alto índice de desbalance nutrimental (IDN), coeficiente de transferencia, la relación P/Fe y K/Ca, concentración de K, Ca, Mg, Mn, Zn, Cu y B en hojas jóvenes de frijol en hojas con clorosis férrica. En ausencia de Fe la línea 496 presentó menos clorosis, incrementó la relación P/Mg y la concentración de P y K. Cuando el Fe estuvo presente en la solución nutritiva, las líneas 496 y 33 tuvieron índices nutrimentales bajos y mayor producción de materia seca. La línea T2 fue susceptible a clorosis férrica, pero con una concentración de 1 mg L-1 de Fe en la solución nutritiva, presentó mayor producción de materia seca, volumen radical y no manifestó clorosis férrica. La línea 33 fue susceptible y en ausencia de Fe en la solución, aumentó las relaciones N/P, B/P, Ca/P y disminuyó la concentración de P, K y B. La adición de 1 mg L-1 de Fe en la solución nutritiva aumentó la concentración de N, P, K y Fe, mientras que en ausencia incrementó la concentración de Mn, Zn y Cu en raíz. Las diferencias encontradas en la clorosis férrica de frijol en plantas tolerantes y susceptibles no obedecen a la concentración de Fe sino a mecanismos internos, relacionados con otros elementos minerales que afectan su metabolismo.
Descargas
Citas
Abadía, B. A.; Sanz, E. M.; Rivas, J. and Abadía, B. J. 1989. Photosynthetic pigments and mineral composition of iron deficient pear leaves. J. Plant Nutr. 12(7):827-838.
Abadía, B. J. 1992. Leaf responses to Fe deficiency: a review. J. Plant Nutr. 15(10):1699-1713.
Abadía, B. J. and Abadía, B. A. 1993. Iron and plant pigments. In: iron chelation in plants and soil microorganisms. Ed. Academic press, New York. 327-343 pp.
Abadía, B. J.; Morales, I. F. and Abadía, B. A. 2000. Photosystem II efficiency in low chlorophyll, iron-deficiency leaves. Plant and Soil. 215(1):183-192.
Alhendawi, R. A.; Volker, R.; Kirkby, E. A. and Marschner, H. 1997. Influence of increasing bicarbonate concentrations on plant growth, organic acid accumulation in roots and iron uptake by barley, sorghum, and maize. J. Plant Nutr. 20(12):1731-1753.
Arahou, M. and Diem, H. G. 1997. Iron deficiency induce cluster (proteoid) root formation in Casuarina glauca. Plant and Soil. 196(1):71-79.
Belkhodja, R. F; Morales, I. F.; Sanz, E. M.; Abadía, B. A. and Abadía, B. J. 1998. Iron deficiency in peach trees: effects on leaf chlorophyll and nutrient concentrations in flowers and leaves. Plant and Soil. 203(1):257-268.
Bertamini, M. and Nedunchzhin, N. 2005. Grapevine growth and physiological response to iron deficiency. J. Plant Nutr. 28(5):157-163.
Bohórquez, J. M.; Romera, F. J. Alcántara, V. E. 2001. Effect of Fe3+, Zn2+ and Mn2+ on ferric reducing capacity and regreening process of the peach rootstock Nemaguard (Prunus persica (L.) Batsch). Plant and Soil. 237(1):157-163.
Campbell, S. A. and Nishio, J. N. 2000. Iron deficiency studies of sugar beet using an improved sodium bicarbonate-buffered hydroponic growth system. J. Plant Nutr. 23(6):741-757.
Clark, R. B. 1991. Iron: unlocking agronomic potential. Solutions. 35(3):24-28.
Connorton, J. M.; Balk, J. and Rodríguez, C. J. 2017.Iron homeostasis in plants an overview in. Metallomics. 9(7):813-823.
Cramer, M. D. O.; Lewis, A. M. and Lips, S. H. 1993. Inorganic carbon fixation and metabolism in maize roots as affected by nitrate and ammonium nutrition. Physiol. Plant. 89(3):632-639.
Curtin, C. H. and Wen, G. Z. 2004. Plant cation-anion balance as affected by the ionic composition of the growing medium. Plant Soil. 267(1/2):109-115.
Dell’Orto, M. X.; Nisi, P. D.; Pontiggia, A. and Zocchi, G. 2003. Fe deficiency response in Parietaria diffusa: a calcicole plant. J. Plant Nutr. 26(10):2057-268.
Foy, C. D. M.; Farina, P. W. and Oakes, A. J. 1998. Iron manganese interactions among clones of nilegrass. J. Plant Nutr. 21(5):987-1009.
Jia, X. M.; Zhu, Y. F.; Hu, Y.; Cheng, L.; Zhao, T. and Wang, Y. 2018. Tolerance to iron-deficiency stress of three apple rootstock species in hydroponic system. Agric. Sci. Technol. 19(1):21-30.
Kassas, S. E. 1984. Effect of iron nutrition on the growth, yield, fruit quality and leaf composition of seeded balady lime stress grown on sandy calcareous soil. J. Plant Nutr. 11(1-5):677-690.
Keshirad, A. A.; Bassiri, A. and Kheradnan, M. 1978. Responses of cowpeas to applications of P and Fe in calcareous soils. Agron. J. 70(1):67-70.
Kosegarten, H.U.; Hoffmann, B. and Mengel K. 1999. Apoplastic pH and Fe3+ reduction in intact sunflower leaves. Plant Physiol. 121(4):1069-1079.
Köseoglu, A. T. 1995. Effect of iron chlorosis on mineral composition of peach leaves. J. Plant Nutr. 18(4):765-776.
Landsberg, E. C. 1996. Hormonal regulation of iron-stress response in sunflower roots: a morphological and cytological investigation. Protoplasma. 194(1):60-80.
Li, J.; Cao, X.; Jia, X. Liu, L.; Cao, H.; Qin, H. and Li, M. 2021. Iron deficiency leads to chlorosis through impacting chlorophyll synthesis and nitrogen metabolism in Areca Catechu L. Front Plant Sci. 12(710093):1-17.
López-Millán, Ana-Flor; Grusak, M. A; Abadía B. A. and Abadía B. J. 2013. Iron deficiency in plants: an insight from proteomic approaches. Front. Plant Sci. 4 (254) 1-7.
Loué, A. 1988. Los micronutrimentos en la agricultura. Traductor: Domínguez, A. V. Mundi-Prensa. Madrid, España. 200-354 pp.
Maldonado, T. R.; Etchevers, B. J. D.; Alcántar, G. J.; Rodríguez, A. J. and Colinas, L. M. T. 2006. Morphological changes in leaves of Mexican lime affected by iron chlorosis. J. Plant Nutr. 29(4):615-628.
Marika, B.; Maria, L. B.; Simona, C.; Tanja, M.; Stefano, C.; Youry, P.; Emidio, A. and Daniele, D.B. 2015. Iron deficiency in barley plants: phytosiderophore release, iron translocation, and DNA methylation. Front. Plant Sci. 6(514):1-12.
Marschner, H. 1988. Mechanisms of manganese acquisition by roots from soils. In: manganese in soils and plants. (Ed). Kluwer academic publishers. Boston. 191-204 pp.
Marschner, H. 1991. Symposium summary and future research areas. Ed. Iron nutrition and interaction in plants. Kluwer academic publisher. The Netherlands. 365-372 pp.
Marschner, H. 1995. Mineral nutrition of higher plants. Academic press. London. 313-324 pp.
Morales, I. F.; Grasa, R.; Abadía, B. A. and Abadía, B. J. 1998. Iron chlorosis paradox in fruit trees. J. Plant Nutr. 21(4):815-825.
Parrilla, L. and Schmidt, W. 2019. Iron acquisition strategies in land plants: not so different after all. New Phytologist. 224(1):11-18.
Rijck, C. and Schrevens, E. 1997. Ph influenced by the elemental composition of nutrient solutions. J. Plant Nutr. 20(7-8):911-923.
Riaz, N. and Guerinot, M. L. 2021. All together now: regulation of the iron deficiency response. J. Exp. Bot. 72(6):2045-2055.
Robinson, N. J.; Procter, C. M.; Connolly, E. L. and Guerinot, M. L. 1999. A ferric-chelate reductase for iron uptake from soils. Nature. 397(6721):694-697.
Römheld, V. 2000. The chlorosis paradox: Fe inactivation as a secondary event in chlorotic leaves of grapevine. J. Plant Nutr. 23(11-12):1629-1643
Rout, G. R. and Sunita, S. 2015. Role of iron in plant growth and metabolism. Reviews Agric. Sci. 3(1-2):1-24.
Schaller, G. 1987. pH changes in the rhizosphere in relation to the pH-buffering of soils. Plant Soil. 97(3):439-444.
Schmidt, W. and Bartels, M. 1996. Formation of root epidermal transfer cell in Plantago. Plant Physiol. 110(1):217-225.
Schmidt, W.; Thomine, S. and Buckhout, T. J. 2020. Iron nutrition and interactions in plants. Front. Plant Sci. 10(670)1-4.
Susin, S. A.; Abadía, J. A.; González-Reyes, J.; Lucena, J. J. and Abadía, B. J. 1996. The pH requirements for in vivo activity of the iron deficiency-induced ‘Turbo’ ferric chelate reductase: a comparation of the iron deficiency induced iron reductase activities of intact plants and isolated plasma membrane fractions in sugar beet. Plant Physiol. 110(1):111-123.
Thomas, F. M.; Brandt, T. and Hartmann, G. 1998. Leaf chlorosis in Pedunculate oaks (Quercus robur L.) on calcareous soils resulting from lime induced manganese/iron deficiency: soil conditions and physiological reactions. Angew. Bot. 72(1-2):28-36.
Toulon, V.; Sentenac H.; Thibaud, J.B.; Davidian, J. C.; Moulineau, C. and Grignon, C. 1992. Role of apoplastic acidification by H+ pump: effect on the sensitivity to pH and CO2 of iron reduction by roots of Brassica napus L. Planta. 186(2):212-218.
Tripathi, D. K.; Singh, S.; Gaur, S.; Singh, S.; Yadav, V.; Liu, S.; Singh; Sharma, S.; Srivastava, P.; Prasad; S.; Dubey; N. K.; Chauhan, D. K. and Sahi, S. 2018. Acquisition and homeostasis of iron in higher plants and their probable role in abiotic stress tolerance. Front. Environ. Sci. 5(86):1-15.
Wairich, A.; Oliveira, B. N.; Arend, E. B.; Duarte, G. L.; Ponte, L. R.; Sperotto, R. A.; Ricachenevsky, F. K. and Fett, J. P. 2019. The combined strategy for iron uptake is not exclusive to domesticated rice (Oryza sativa). Scientific Reports. 9(16144):1-17.
Welkie, G. W. and Miller, G. W. 1993. Plant iron uptake physiology by nonsiderophore system. In: iron chelation in plants and soils microorganisms. Ed. Barton, L. L. and Hemming, B. C. Academic press. New York. 345-370 pp.
Yang, X.; Römheld, V. and Marschner, H. 1994. Effect of bicarbonate on root growth and accumulation of organic acids in Zn-inefficient and efficient rice cultivars Oriza sativa L. Plant and Soil. 164(1):1-7.
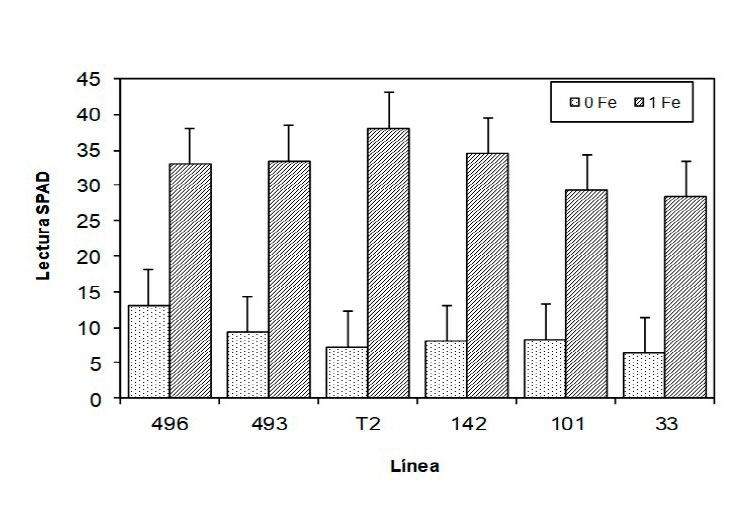
Descargas
Publicado
Cómo citar
Número
Sección
Licencia
Derechos de autor 2023 Revista Mexicana de Ciencias Agrícolas

Esta obra está bajo una licencia internacional Creative Commons Atribución-NoComercial 4.0.
Los autores(as) que publiquen en Revista Mexicana de Ciencias Agrícolas aceptan las siguientes condiciones:
De acuerdo con la legislación de derechos de autor, Revista Mexicana de Ciencias Agrícolas reconoce y respeta el derecho moral de los autores(as), así como la titularidad del derecho patrimonial, el cual será cedido a la revista para su difusión en acceso abierto.
Los autores(as) deben de pagar una cuota por recepción de artículos antes de pasar por dictamen editorial. En caso de que la colaboración sea aceptada, el autor debe de parar la traducción de su texto al inglés.
Todos los textos publicados por Revista Mexicana de Ciencias Agrícolas -sin excepción- se distribuyen amparados bajo la licencia Creative Commons 4.0 atribución-no comercial (CC BY-NC 4.0 internacional), que permite a terceros utilizar lo publicado siempre que mencionen la autoría del trabajo y a la primera publicación en esta revista.
Los autores/as pueden realizar otros acuerdos contractuales independientes y adicionales para la distribución no exclusiva de la versión del artículo publicado en Revista Mexicana de Ciencias Agrícolas (por ejemplo incluirlo en un repositorio institucional o darlo a conocer en otros medios en papel o electrónicos) siempre que indique clara y explícitamente que el trabajo se publicó por primera vez en Revista Mexicana de Ciencias Agrícolas.
Para todo lo anterior, los autores(as) deben remitir el formato de carta-cesión de la propiedad de los derechos de la primera publicación debidamente requisitado y firmado por los autores(as). Este formato debe ser remitido en archivo PDF al correo: revista_atm@yahoo.com.mx; revistaagricola@inifap.gob.mx.
Esta obra está bajo una licencia de Creative Commons Reconocimiento-No Comercial 4.0 Internacional.