Pseudomonas spp. beneficial in agriculture
DOI:
https://doi.org/10.29312/remexca.v13i4.2799Keywords:
beneficial microorganisms, biocontrol, biofertilizers, suppressive soilsAbstract
Bacteria of the genus Pseudomonas inhabit a wide variety of environments, which is a reflection of their diverse metabolic capacity, this has allowed them to adapt to variable environmental conditions, also, this genus is considered ambivalent due to the fact that some species establish beneficial relationships with plants and others pathogenic relationships with plants, animals and humans. In the present work, we focus on the positive impact that this bacterial genus has in the agricultural field, due to its capacity as a plant growth-promoting bacterium (PGPB), being one of the best options as a plant and soil inoculant, to improve plant growth and disease management, through the wide range of metabolites that the beneficial strains are capable of producing, bacteria of this genus have been identified with diazotrophic capacity, producing antibiotics, auxins, siderophores, cellulolytic enzymes, organic acids for phosphorus solubilization and promotion of induced systemic resistance against phytopathogens, which makes them ideal in agricultural production either for biocontrol or biofertilization, likewise, their use does not affect the environment or the health of farmers.
Downloads
References
Aiyar, P.; Schaeme, D.; García-Altares, M.; Flores, D. C.; Dathe, H.; Hertweck, C. and Mittag, M. 2017. Antagonistic bacteria disrupt calcium homeostasis and immobilize algal cells. Nature Comm. 8(1):1-13. https://doi.org/10.1038/s41467-017-01547-8. DOI: https://doi.org/10.1038/s41467-017-01547-8
Baker, K. F. and CooK, R. J. 1991. Biological control of plant pathogens. San Francisco: W/H Freeman & Co. Millington, S. 28-29 pp.
Biessy, A. and Filion, M. 2018. Phenazines in plant‐beneficial Pseudomonas spp.: biosynthesis, regulation, function, and genomics. Environ Microbiol. 20(11):3905-3917. https://doi.org/ 10.1111/1462-2920.14395.
Bender, C. L.; Rangaswamy, V. and Loper, J. 1999. Polyketide production by plant-associated pseudomonads. Annual Review Phytopathol. 37(1):175-196. https://doi.org/10.1146/ annurev.phyto.37.1.175. DOI: https://doi.org/10.1146/annurev.phyto.37.1.175
Chin‐A‐Woeng, T. F.; Bloemberg, G. V. and Lugtenberg, B. J. 2003. Phenazines and their role in biocontrol by Pseudomonas bacteria. New Phytologist. 157(3):503-523. https://doi.org/10. 1046/j.1469-8137.2003.00686.x DOI: https://doi.org/10.1046/j.1469-8137.2003.00686.x
Geudens, N. and Martins, J. C. 2018. Cyclic lipodepsipeptides from Pseudomonas spp.-biological swiss-army knives. Frontiers Microbiol. 9:1867. https://doi.org/10.3389/fmicb. 2018.01867.
Gross, H. and Loper, J. E. 2009. Genomics of secondary metabolite production by Pseudomonas spp. Natural Product Reports. 26(11):1408-1446. https://doi.org/10.1039/b817075b. DOI: https://doi.org/10.1039/b817075b
Gutiérrez-García, K.; Neira-González, A.; Pérez-Gutiérrez, R. M.; Granados-Ramírez, G.; Zarraga, R., Wrobel, K. and Flores-Cotera, L. B. 2017. Phylogenomics of 2-4. Diacetylphloroglucinol-producing pseudomonas and novel antiglycation endophytes from piper Auritum. J. Natur. Produc. 80(7):1955-1963. https://doi.org/10.1021/acs.jnatprod. 6b00823. DOI: https://doi.org/10.1021/acs.jnatprod.6b00823
Guttenberger, N.; Blankenfeldt, W. and Breinbauer, R. 2017. Recent developments in the isolation, biological function, biosynthesis, and synthesis of phenazine natural products. Bioo. Med. Chem. 25(22):6149-6166. https://doi.org/10.1016/j.bmc.2017.01.002. DOI: https://doi.org/10.1016/j.bmc.2017.01.002
Haas, D. and Keel, C. 2003. Regulation of antibiotic production in root colonizing Pseudomonas spp. and relevance for biological control of plant disease. Annu. Rev. Phytopathol. 41(1):117-153. https://doi.org/10.1146/annurev.phyto.41.052002.095656. DOI: https://doi.org/10.1146/annurev.phyto.41.052002.095656
Jara, H. A. D. y Elizondo E. A. M. 2011. Suelos supresivos a enfermedades radicales: ‘declinación del mal de pie (Gaeumannomyces graminis var. tritici) en trigo’, un estudio de caso. Agro Sur. 39(2):67-78. https://doi.org/10.4206/agrosur.2011.v39n2-01. DOI: https://doi.org/10.4206/agrosur.2011.v39n2-01
Jang, J. Y.; Yang, S. Y.; Kim, Y. C.; Lee, C. W.; Park, M. S.; Kim, J. C. and Kim, I. S. 2013. Identification of orfamide A as an insecticidal metabolite produced by pseudomonas protegens F6. J. Agric. Food Chem. 61(28):6786-6791. https://doi.org/10.1021/jf401218w. DOI: https://doi.org/10.1021/jf401218w
Julian, W. T.; Vasilchenko, A. V.; Shpindyuk, D. D.; Poshvina, D. V. and Vasilchenko, A. S. 2021. Bacterial-derived plant protection metabolite 2, 4-diacetylphloroglucinol: effects on bacterial cells at inhibitory and subinhibitory concentrations. Biomolecules. 11(1):13. https://doi.org/10.3390/biom11010013.
Kankariya, R. A.; Chaudhari, A. B.; Gavit, P. M. and Dandi, N. D. 2019. 2-4. Diacetylphloroglucinol: a novel biotech bioactive compound for agriculture. In microbial interventions in agriculture and environment. Springer, Singapore. 419-452 pp. https://doi.org/10.1007/978-981-13-8391-5-16.
Keswani, C.; Singh, H. B.; García-Estrada, C.; Caradus, J.; He, Y. W.; Mezaache-Aichour, S. and Sansinenea, E. 2020. Antimicrobial secondary metabolites from agriculturally important bacteria as next-generation pesticides. Appl. Microbiol. Biotechnol. 104(3):1013-1034. https://doi.org/10.1007/s00253-019-10300-8.
Keel, C.; Oberhansli, T.; Wirthner, P.; Voisard, C.; Haas, D. and Défago, G. 1990. Pseudomonads as antagonists of plant pathogens in the rhizosphere: role of the antibiotic 2-4, diacetylphloroglucinol in the suppression of black root rot of tobacco. Symbiosis.
Kumar, A.; Verma, H.; Singh, V. K.; Singh, P. P.; Singh, S. K.; Ansari, W. A. and Pandey, K. D. 2017. Role of Pseudomonas sp. in sustainable agriculture and disease management. In: agriculturally important microbes for sustainable agriculture. Springer, Singapore. 195-215 pp. https://doi.org/10.1007/978-981-10-5343-6-7. DOI: https://doi.org/10.1007/978-981-10-5343-6_7
Khan, H.; Parmar, N. and Kahlon, R. S. 2016. Pseudomonas-plant interactions I: plant growth promotion and defense-mediated mechanisms. In: Pseudomonas: molecular and applied biology. Springer, Cham. 419-468 pp. https://doi.org/10.1007/978-3-319-31198-2-10. DOI: https://doi.org/10.1007/978-3-319-31198-2_10
Kwak, Y. S.; Han, S.; Thomashow, L. S.; Rice, J. T.; Paulitz, T. C.; Kim, D. and Weller, D. M. 2011. Saccharomyces cerevisiae genome-wide mutant screen for sensitivity to 2, 4-diacetylphloroglucinol, an antibiotic produced by Pseudomonas fluorescens. Appl. Environ. Microbiol. 77(5):1770-1776. https://doi.org/10.1128/aem.02151-10. DOI: https://doi.org/10.1128/AEM.02151-10
Lambowitz, A. M. and Slayman, C. W. 1972. Effect of pyrrolnitrin on electron transport and oxidative phosphorylation in mitochondria isolated from Neurospora crassa. J. Bacteriol. 112(2):1020-1022. https://doi.org/10.1128/jb.112.2.1020-1022.1972.
Ligon, J. M.; Hill, D. S.; Hammer, P. E.; Torkewitz, N. R.; Hofmann, D.; Kempf, H. J. and Pée, K. H. V. 2000. Natural products with antifungal activity from Pseudomonas biocontrol bacteria. Pest Management Science: formerly Pesticide Science. 56(8):688-695. https://doi.org/10.1533/9781845698416.4.179. DOI: https://doi.org/10.1002/1526-4998(200008)56:8<688::AID-PS186>3.0.CO;2-V
Loper, J. E.; Henkels, M. D.; Rangel, L. I.; Olcott, M. H.; Walker, F. L.; Bond, K. L. and Taylor, B. J. 2016. Rhizoxin analogs, orfamide A and chitinase production contribute to the toxicity of Pseudomonas protegens strain Pf‐5 to Drosophila melanogaster. Environ. Microbiol. 18(10):3509-3521. https://doi.org/10.1111/1462-2920.13369. DOI: https://doi.org/10.1111/1462-2920.13369
Lugtenberg, B. J. J.; De Weger, L. A. and Schippers, B. 1994. Bacterization to protect seed and rhizosphere against disease. BCPC Monograph. 57:293-302.
Lugtenberg, B. J.; Kravchenko, L. V. and Simons, M. 1999. Tomato seed and root exudate sugars: composition, utilization by Pseudomonas biocontrol strains and role in rhizosphere colonization. Environ. Microbiol. 1(5):439-446. https://doi.org/10.1046/j.1462-2920.1999. 00054.x. DOI: https://doi.org/10.1046/j.1462-2920.1999.00054.x
Ma, Z.; Geudens, N.; Kieu, N. P.; Sinnaeve, D.; Ongena, M.; Martins, J. C. and Höfte, M. 2016. Biosynthesis, chemical structure, and structure-activity relationship of orfamide lipopeptides produced by Pseudomonas protegens and related species. Frontiers Microbiol. 7:382. https://doi.org/10.3389/fmicb.2016.00382. DOI: https://doi.org/10.3389/fmicb.2016.00382
Ma, Z.; Ongena, M. and Höfte, M. 2017. The cyclic lipopeptide orfamide induces systemic resistance in rice to Cochliobolus miyabeanus but not to Magnaporthe oryzae. Plant Cell Reports. 36(11):1731-1746. https://doi.org/10.1007/s00299-017-2187-z. DOI: https://doi.org/10.1007/s00299-017-2187-z
Malviya, D.; Sahu, P. K.; Singh, U. B.; Paul, S.; Gupta, A.; Gupta, A. R. and Brahmaprakash, G. P. 2020. Lesson from Ecotoxicity: revisiting the microbial lipopeptides for the management of emerging diseases for crop protection. Inter. J. Environ. Res. Public Health. 17(4):1434. https://doi.org/10.3390/ijerph17041434.
Mavrodi, D. V.; Blankenfeldt, W. and Thomashow, L. S. 2006. Phenazine compounds in fluorescent Pseudomonas spp. biosynthesis and regulation. Annu. Rev. Phytopathol. 44(1):417-445. https://doi.org/10.1146/annurev.phyto.44.013106.145710. DOI: https://doi.org/10.1146/annurev.phyto.44.013106.145710
National Center for Biotechnology Information. 2021. PubChem Compound. https://pubchem.ncbi.nlm.nih.gov/.
Neiendam, N. M. and Sørensen, J. 1999. Chitinolytic activity of Pseudomonas fluorescens isolates from barley and sugar beet rhizosphere. FEMS Microbiol. Ecol. 30(3):217-227. https://doi.org/10.1111/j.1574-6941.1999.tb00650.x. DOI: https://doi.org/10.1111/j.1574-6941.1999.tb00650.x
Nose, M. and Arima, K. 1969. On the mode of action of a new antifungal antibiotic, pyrrolnitrin. The J. Antibi. 22(4):135-143. https://doi.org/10.7164/antibiotics.22.135. DOI: https://doi.org/10.7164/antibiotics.22.135
Pawar, S.; Chaudhari, A.; Prabha, R.; Shukla, R. and Singh, D. P. 2019. Microbial pyrrolnitrin: natural metabolite with immense practical utility. Biomolecules. 9(9):443. https://doi.org/10.3390/biom9090443.
Pierson, L. S. and Thomashow, L. S. 1992. Cloning and heterologous expression of the phenazine biosynthetic. Mol. Plant-Microbe Interact. 5(4):330-339. https://doi.org/10.1094/mpmi-5-330. DOI: https://doi.org/10.1094/MPMI-5-330
Pieterse, C. M.; Van Pelt, J. A.; Van Wees, S. C.; Ton, J.; Léon-Kloosterziel, K. M.; Keurentjes, J. J. and Van Loon, L. C. 2001. Rhizobacteria-mediated induced systemic resistance: triggering, signalling and expression. Eur. J. Plant Pathol. 107(1):51-61. https://doi.org/10.1023/a:1008747926678. DOI: https://doi.org/10.1023/A:1008747926678
Preston, G. M. 2004. Plant perceptions of plant growth-promoting Pseudomonas. Philosophical Transactions of the Royal Society of London. Series B. Biol. Sci. 359(1446):907-918. https://doi.org/10.1098/rstb.2003.1384. DOI: https://doi.org/10.1098/rstb.2003.1384
Quan, C. S.; Wang, X. and Fan, S. D. 2010. Antifungal compounds of plant growth promoting rhizobacteria and its action mode. In: plant growth and health promoting bacteria. Springer, Berlin, Heidelberg. 117-156 pp. https://doi.org/10.1007/978-3-642-13612-2-6. DOI: https://doi.org/10.1007/978-3-642-13612-2_6
Raaijmakers, J. M.; De Bruijn, I. and de Kock, M. J. 2006. Cyclic lipopeptide production by plant-associated Pseudomonas spp.: diversity, activity, biosynthesis, and regulation. Mol. Plant-Microbe Interactions. 19(7):699-710. https://doi.org/10.1094/mpmi-19-0699. DOI: https://doi.org/10.1094/MPMI-19-0699
Ramette, A.; Frapolli, M.; Fischer-Le Saux, M.; Gruffaz, C.; Meyer, J. M.; Défago, G.; Sutra, L. and Moënne-Loccoz, Y. 2011. Pseudomonas protegens sp. nov., widespread plant-protecting bacteria producing the biocontrol compounds 2,4-diacetylphloroglucinol and pyoluteorin. System. Appl. Microbiol. 34(3):180-188. https://doi.org/10.1016/j.syapm. 2010.10.005. DOI: https://doi.org/10.1016/j.syapm.2010.10.005
Ran, L. X.; Li, Z. N.; Wu, G. J.; Van Loon, L. C. and Bakker, P. A. H. M. 2005. Induction of systemic resistance against bacterial wilt in Eucalyptus urophylla by fluorescent Pseudomonas spp. Eur. J. Plant Pathol. 113(1):59-70. https://doi.org/10.1007/s10658-005-0623-3. DOI: https://doi.org/10.1007/s10658-005-0623-3
Rathinasabapathi, B.; Liu, X.; Cao, Y. and Ma, L. Q. 2018. Phosphate-solubilizing Pseudomonads for improving crop plant nutrition and agricultural productivity. In: Crop Improvement Through Microbial Biotechnology. Elsevier. 363-372 pp. https://doi.org/10.1016/b978-0-444-63987-5.00018-9. DOI: https://doi.org/10.1016/B978-0-444-63987-5.00018-9
Rhodes, D. J. and Powell, K. A. 1994. Biological seed treatments the development process. BCPC Monograph. 57:303-310.
Sah, S. and Singh, R. 2015. Siderophore: structural and functional characterisation a comprehensive review. Agriculture Pol’nohospodárstvo. 61(3):97-114. https://doi.org/ 10.1515/agri-2015-0015. DOI: https://doi.org/10.1515/agri-2015-0015
Schwanemann, T.; Otto, M.; Wierckx, N. and Wynands, B. 2020. Pseudomonas as versatile aromatics cell factory. Biotechnol. J. 15(11):1900569. https://doi.org/10.1002/biot. 201900569.
Smirnov, V. A. and Kiprianova, E. A. 1990. Bacteria of Pseudomonas genus. Kiev. Naukova Dumka. 264 p.
Thomashow, L. S. 2013. Phenazines in the environment: microbes, habitats, and ecological relevance. In Microbial phenazines. Springer, Berlin, Heidelberg. 199-216 pp. https://doi.org/10.1007/978-3-642-40573-0-10. DOI: https://doi.org/10.1007/978-3-642-40573-0_10
Troppens, D. M.; Chu, M.; Holcombe, L. J.; Gleeson, O.; O’Gara, F.; Read, N. D. and Morrissey, J. P. 2013. The bacterial secondary metabolite 2, 4-diacetylphloroglucinol impairs mitochondrial function and affects calcium homeostasis in Neurospora crassa. Fungal Genetics and Biology. 56:135-146. https://doi.org/10.1016/j.fgb.2013.04.006. DOI: https://doi.org/10.1016/j.fgb.2013.04.006
Turner, J. M. and Messenger, A. J. 1986. Occurrence, biochemistry and physiology of phenazine pigment production. Adv. Microbial Physiol. 27:211-275. https://doi.org/10.1016/ s0065-2911(08)60306-9. DOI: https://doi.org/10.1016/S0065-2911(08)60306-9
Van Loon, L. C.; Bakker, P. A. H. M. and Pieterse, C. M. J. 1998. Systemic resistance induced by rhizosphere bacteria. Ann Review Phytopathol. 36(1):453-483. https://doi.org/10.1146/ annurev.phyto.36.1.453. DOI: https://doi.org/10.1146/annurev.phyto.36.1.453
Van Peer, R. and Schippers, B. 1992. Lipopolysaccharides of plant-growth promoting Pseudomonas sp. strain WCS417r induce resistance in carnation to Fusarium wilt. Netherlands J. Plant Pathol. 98(2):129-139. https://doi.org/10.1007/bf01996325. DOI: https://doi.org/10.1007/BF01996325
Weller, D. M.; Landa, B. B.; Mavrodi, O. V.; Schroeder, K. L.; De La Fuente, L.; Blouin, B. S. and Thomashow, L. S. 2007. Role of 2, 4‐diacetylphloroglucinol‐producing fluorescent Pseudomonas spp. in the defense of plant roots. Plant Biol. 9(1):4-20. https://doi.org/10.1055/s-2006-924473. DOI: https://doi.org/10.1055/s-2006-924473
Weller, D. M.; Raaijmakers, J. M.; Gardener, B. B. M. and Thomashow, L. S. 2002. Microbial populations responsible for specific soil suppressiveness to plant pathogens. Ann. Review Phytopathol. 40(1):309-348. https://doi.org/10.1146/annurev.phyto.40.030402.110010. DOI: https://doi.org/10.1146/annurev.phyto.40.030402.110010
Wendenbaum, S.; Demange, P.; Dell, A.; Meyer, J. M. and Abdallah, M. A. 1983. The structure of pyoverdine Pa, the siderophore of Pseudomonas aeruginosa. Tetrahedron Letters. 24(44):4877-4880. https://doi.org/10.1016/s0040-4039(00)94031-0. DOI: https://doi.org/10.1016/S0040-4039(00)94031-0
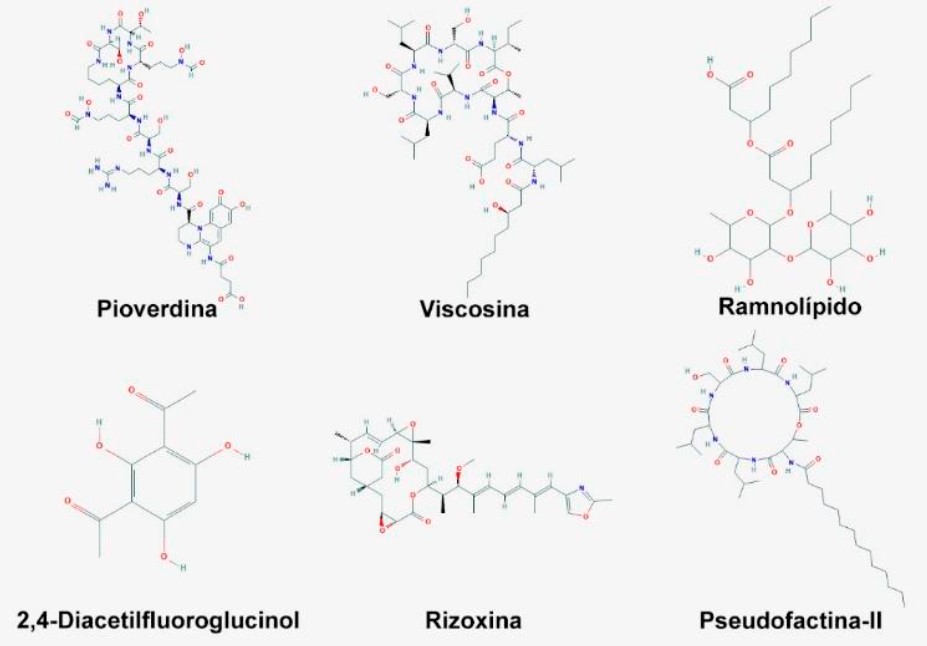
Published
How to Cite
Issue
Section
License
Copyright (c) 2022 Revista Mexicana de Ciencias Agrícolas

This work is licensed under a Creative Commons Attribution-NonCommercial 4.0 International License.
The authors who publish in Revista Mexicana de Ciencias Agrícolas accept the following conditions:
In accordance with copyright laws, Revista Mexicana de Ciencias Agrícolas recognizes and respects the authors’ moral right and ownership of property rights which will be transferred to the journal for dissemination in open access. Invariably, all the authors have to sign a letter of transfer of property rights and of originality of the article to Instituto Nacional de Investigaciones Forestales, Agrícolas y Pecuarias (INIFAP) [National Institute of Forestry, Agricultural and Livestock Research]. The author(s) must pay a fee for the reception of articles before proceeding to editorial review.
All the texts published by Revista Mexicana de Ciencias Agrícolas —with no exception— are distributed under a Creative Commons License Attribution-NonCommercial 4.0 International (CC BY-NC 4.0), which allows third parties to use the publication as long as the work’s authorship and its first publication in this journal are mentioned.
The author(s) can enter into independent and additional contractual agreements for the nonexclusive distribution of the version of the article published in Revista Mexicana de Ciencias Agrícolas (for example include it into an institutional repository or publish it in a book) as long as it is clearly and explicitly indicated that the work was published for the first time in Revista Mexicana de Ciencias Agrícolas.
For all the above, the authors shall send the Letter-transfer of Property Rights for the first publication duly filled in and signed by the author(s). This form must be sent as a PDF file to: revista_atm@yahoo.com.mx; cienciasagricola@inifap.gob.mx; remexca2017@gmail.
This work is licensed under a Creative Commons Attribution-Noncommercial 4.0 International license.